Coffee arabica-derived copper nanoparticles: A potent larvicidal agent against Aedes aegypti mosquitoes
Abstract
Mosquito-borne diseases, transmitted by Aedes aegypti mosquitoes, are a major public health concern. This study aimed to examine the larvicidal activity of Coffee arabica-mediated copper nanoparticles (CuNPs) against Aedes aegypti mosquito larvae. CuNPs were synthesized from Coffee arabica extract and characterized using advanced techniques such as UV spectroscopy, FTIR, and X-ray diffraction. The extract contained bioactive compounds with antioxidant properties. Green coffee had higher total phenolic content and total flavonoid capacity than roasted coffee. Green coffee extract exhibited stronger antioxidant activity and lower inhibitory concentration (IC50) values compared to roasted coffee extract. The larvicidal effects of green and roasted Coffee arabica extracts were assessed. Green Coffee arabica had lethal concentration (LC50) and LC90 of 124.5 ppm and 456.5 ppm, respectively, while roasted Coffee arabica had LC50 and LC90 of 73.5 ppm and 371.5 ppm, respectively. CuNPs at concentrations of 2 to 100 ppm resulted in LC50 and LC90 of 5.796 ppm and 36.595 ppm, respectively. The synthesized CuNPs demonstrated concentration-dependent mortality, with lower LC50 values compared to coffee extracts alone. Histological analysis showed tissue degradation in treated larvae, particularly in the head region. CuNPs had a detrimental effect on Artemia salina larvae, with higher concentrations leading to increased mortality. The LC50 and LC90 values for Artemia salina were determined as 344.3 ppm and 1073.2 ppm, respectively. Overall, Coffee arabica-mediated CuNPs displayed potent larvicidal activity against Aedes aegypti mosquitoes, indicating their potential use in eco-friendly mosquito control strategies.
INTRODUCTION
The use of green biological chemistry has made it easy, cheap, and quick to create metallic nanoparticles such as copper, silver, gold, and selenium in recent years. The synthesis of these nanoparticles was done in a manner that was clean, non-toxic, and environment-friendly [1]. Synthesizing nanoparticles from plants is considered relatively faster, as they do not need specialized culture media or microorganism cultivation. In addition, plants contain primary and secondary compounds such as enzymes, proteins, flavonoids, and teraponids that act as reducing and capping agents [2, 3]. Today, nanoparticles are used in various fields of life, such as solar energy, water treatment, medicine, and agriculture, it may be considered a viable solution to challenges that pollute the environment [4, 5]. Biologically synthesized nanoparticles are toxic to harmful bacteria and insects due to their unique properties, including a large surface area that grants high catalytic capacity, facilitating the generation of reactive oxygen species (ROS). These ROS act as potent antimicrobial and insecticidal agents by causing damage to cellular structures and disrupting metabolic processes within target organisms [6, 7].
Many severe infections that cause fever, including dengue, Zika, and Chikungunya are transmitted mostly by mosquitoes [8]. Currently, mosquitoes contribute to the spread of many diseases in urban and rural areas as well. To deal with this problem, scientists recommend using nanotechnology as a solution for diseases transmitted by insects [9]. Green-synthesized metal nanoparticles show promise in controlling insect vectors, including mosquitoes. Copper, gold, palladium, silver, zinc, carbon, and silica nanoparticles have been identified as effective against mosquito populations [10].
Copper is one of the basic elements that a living organism needs physiologically and structurally [11]. Copper nanoparticles (CuNPs) are gaining popularity in various scientific fields due to their low toxicity, high stability, and unique properties. Researchers have also found that biologically synthesized CuNPs are safer, more environment-friendly, and more cost-effective than those produced using chemical or physical methods [12, 13]. A recent report suggests that CuNPs are safe, compatible with living organisms, and free of harmful substances [14]. At the same time, many scientific methods are available to synthesize CuNPs biologically from plant extracts such as dried leaves, seeds, and barks [15-18].
Coffea arabica, a plant belonging to the Rubiaceae family, possesses significant levels of phenolic compounds. These compounds exhibit powerful antioxidant properties and have the ability to hinder the infection caused by severe acute respiratory syndrome coronavirus type 2 [19]. Previous research has shown that an extract derived from C. arabica contains both chlorogenic acid and a small amount of caffeic acid. This extract has been shown to have antioxidant, anti-photoaging, and anti-inflammatory properties in both human skin fibroblasts and mice with no specific genetic background. It also effectively inhibits the production of ROS caused by ultraviolet (UV) radiation [20]. Another research investigation has provided further evidence of the remarkable properties of Coffea arabica extract. In a recent research investigation, the larvicidal activity of Coffea arabica extract was examined. The study found that the extract exhibited potent larvicidal effects against mosquito larvae, specifically those of Aedes aegypti, which is responsible for transmitting diseases such as dengue fever, Zika virus, and chikungunya [21]. Similarly, a research conducted by Kaitana et al. (2022) revealed that the use of Pangi leaf extract is successful in eradicating mosquito larvae and deterring insects [22]. Thus, this study aimed to investigate the potential of Coffee arabica extract synthesized CuNPs for biotoxicity testing against Aedes aegypti larvae and their pathological markers as compared to green and roasted Coffee arabica.
MATERIALS AND METHODS
Chemicals and tools
Coffee arabica seeds were brought from the Bani Hammad area, Taiz, Yemen. Copper sulfate pentahydrate (CuSO4·5H2O) and gallic acid were prepared using the highest-purity materials sourced from Merck, Germany. Additionally, Folin Ciocalteu reagent, aluminum chloride, and Na2CO3 were obtained from WINLAB laboratory chemicals reagent, UK. Na acetate anhydrous, acetic acid, 2,2-diphenyl-1-picrylhydrazyl (DPPH), NaOH, petroleum ether, sulfuric acid, phosphate buffer, as well as reagents such as Benedict's, Biuret's, Phenols, Dragendroff's, Braemer's, Shinoda's, Liebermann's, and Frothing's and methanol were procured from Loba Chemie Pvt Ltd, India. The research employed a spectrophotometer (UNICO 1100-CSO106027) and a centrifuge (800D) (50102002).
Statement on research ethics
The authors obtained ethical clearance from the Animal Ethics Committee at IPB University's Institute of Research and Community Development, Indonesia, with approval number 69-2017 for collecting animal samples in accordance with Indonesia's Regulation (PMK) No. 7 of 2016.
Rearing of target insects
Aedes aegypti mosquito eggs were collected from the colonies reared in laboratories of the Faculty of Medicine, Department of Parasitology, Hasanuddin University, Indonesia. The eggs hatched and turned into larvae under laboratory conditions at room temperature (28°C), humidity and natural lighting. The larvae were kept in non-chlorinated tap water and fed with aquarium fish food.
Plant extract and synthesis of CuNPs
About 20 grams of green Coffee arabica powder and 20 grams of roasted Coffee arabica powder were individually weighed and then combined with 100 ml of distilled water. The mixture was then heated for 1 h at a temperature of 80°C using a magnetic stirrer, and subsequently filtered using ashless filter paper No. 11. 40 (mM) of copper sulfate (CuSO4.5H2O) was prepared and then combined with Coffee arabica extract in a ratio of (1:1). The formation of CuNPs was defined by color change from brown to light green. CuNPs were harvested in powder form after washing it three times with distilled water and centrifugation (HERMLE Z 366 K1) at 12,000 g for 15 minutes, they were put in the furnace to dry for 16 h at 80°C.
Characterization of phytochemicals of Coffee arabica
Qualitative tests were conducted to determine the type of phytochemicals present in Arabic coffee extract according to international protocols [23]. Quantitative tests: the total phenolic content (TPC) of the plant extracts was estimated using the Folin Ciocalteu assay. The assay involved combining Folin Ciocalteu reagent and Na2CO3 with aliquots of the extract or a standard solution of gallic acid. The absorbance was measured at 760 nm after 40 minutes of incubation. A gallic acid curve was used to determine the total phenolic content [24]. The total flavonoid capacity (TFC) was determined using the aluminum chloride (AlCl3) colorimetric method. The extract or a standard quercetin solution was mixed with AlCl3, and the absorbance was measured at 430 nm after 40 minutes. A quercetin plot was used to determine the total flavonoid concentration [25].
Antioxidant potential of Coffee arabica
The antioxidant potential was calculated using the DPPH assay. Control, sample, and blank solutions were prepared, and their absorbance was measured at 517 nm after 30 minutes. The antioxidant activity was determined using the formula of radical scavenging activity (RSA) % = [(A control− A test)/A control] × 100%, and the IC50 value was calculated.
Characterization of CuNPs
The spectrum of CuNPs was studied using a visible/UV spectrophotometer. Wavelengths range from 300 to 800 nm (UV-2600, Shimadzu Europa GmbH). To further characterize the optical properties of CuNPs synthesized from Coffee arabica were analyzed using the X-ray diffusion instrument (Shimadzu XRD-7000). Fourier-transform infrared spectroscopy (FTIR) using an infrared spectrometer (IR Prestige-21, Shimadzu Europa GmbH) was employed to identify the functional groups that contributed to the reduction and stability of CuNPs.
The larvicide bioassay
The parasitic activity was evaluated using the WHO protocol test [26]. Aedes aegypti larvae in the fourth instar were used to assess the effectiveness of CuNPs and Coffee arabica in controlling the parasites. The biotest was conducted in transparent 250 ml cups and repeated three times to ensure the reliability of the statistical outcomes. Each cup contained 20 fresh larvae exposed to different concentrations of CuNPs (2, 12, 25, 50, and 100 ppm), as well as roasted and green Coffee arabica extract (100, 200, 300, 400, and 500 ppm). The results were recorded after 24 h, and the half-lethal concentration (LC50) was determined using probability analysis. The LC50 and LC90 values were calculated using SPSS software, with a significant threshold set at p ≤ 0.05.
Morphological study
The dead larvae of Aedes aegypti treated with CuNPs of Coffee arabica were carefully examined under a microscope (Nikon Microsystems, Japan) to study morphological changes. The outer body parts, including the siphon, setae, eyes, antennae, and anal gills, as well as changes to the abdomen, thorax, and head were carefully monitored. Any visible deviation or discoloration in the body of the treated larvae was recorded and compared to the control group.
Histopathological study
Aedes aegypti larvae were treated with CuNPs of Arabic coffee and the control were fixed with 10 percent buffer formalin for 24 h, then dehydrated, cleared, and sectioned (5 to 7 microns) and stained with hematoxylin-eosin according to a previous study [27].
Ecotoxicology study
The study investigated the effects of green CuNPs on Artemia salina. Brine shrimp eggs hatched and the nauplii were exposed to different concentrations of CuNPs (100, 200, 300, 400 and 500 ppm). After 24 h, the survival rate was calculated using the formula: Mortality Rate = (Number of Deaths) / (Number of Lives) x 100.
Statistical analysis
The statistics software for social sciences SPSS version 25 was used to conduct statistical analysis. Means plus standard deviation were used to represent the data. The single-way analysis of variance (ANOVA) was used to divide variations in the variables into more than two groups, and the variations between the groups were determined.
RESULTS
Production and characterization of CuNPs
CuNPs were produced, leading to a visible shift in color from brown to green. Following this, the nanoparticles underwent characterization using advanced methods including UV spectroscopy, FTIR, and X-ray diffraction. More information about the characterization can be found in our previous research [28]. The average size of CuNPs was measured to be around 16.3 nm phytochemical screening.
Phytochemical profile of Coffee arabica
Table 1 shows the phytochemical content of both green and roasted coffee beans. The roasted coffee beans contained alkaloids, flavonoids, steroids, tannins, phenols, and saponins as phytochemicals. The green coffee samples had similar phytochemicals, except saponins.
Table 1. Coffee arabica metabolites profile.
Total phenolic content and total flavonoid capacity of Coffee arabica
The TPC and TFC of green and roasted coffee are displayed in Figure 1. The TPC and TFC of green coffee were 68.78 ± 0.868 and 21.95 ± 0.331, respectively which are generally higher than those of roasted coffee (65.12 ±0.853 and 19.80±0.259). A statistically significant difference was observed (p < 0.000).
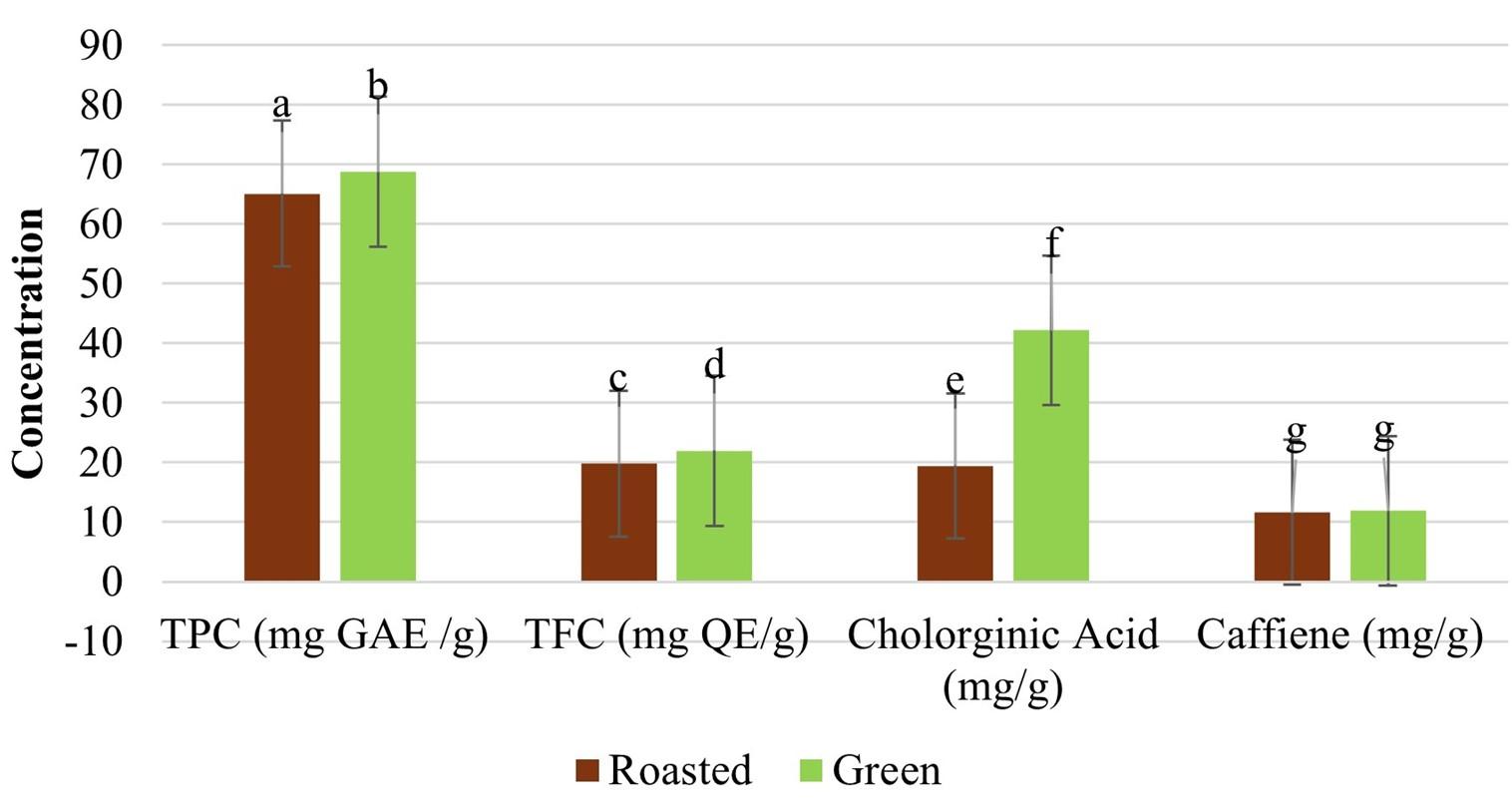
Effect of Coffee arabica-mediated CuNPs on antioxidant potentials
Based on the data presented in Figure 2, it is evident that both roasted and green Coffee arabica extracts exhibited potent free radical scavenging activity. This was supported by high values of DPPH RSA% and low IC50 values, indicating their effectiveness in neutralizing free radicals. Green Coffee arabica extract generally exhibits higher scavenging activity and lower IC50 values (3.37±1.2) compared to roasted Coffee arabica extract (3.21±1.0) at the same concentration. These findings suggest that both extracts have the potential to act as antioxidants.
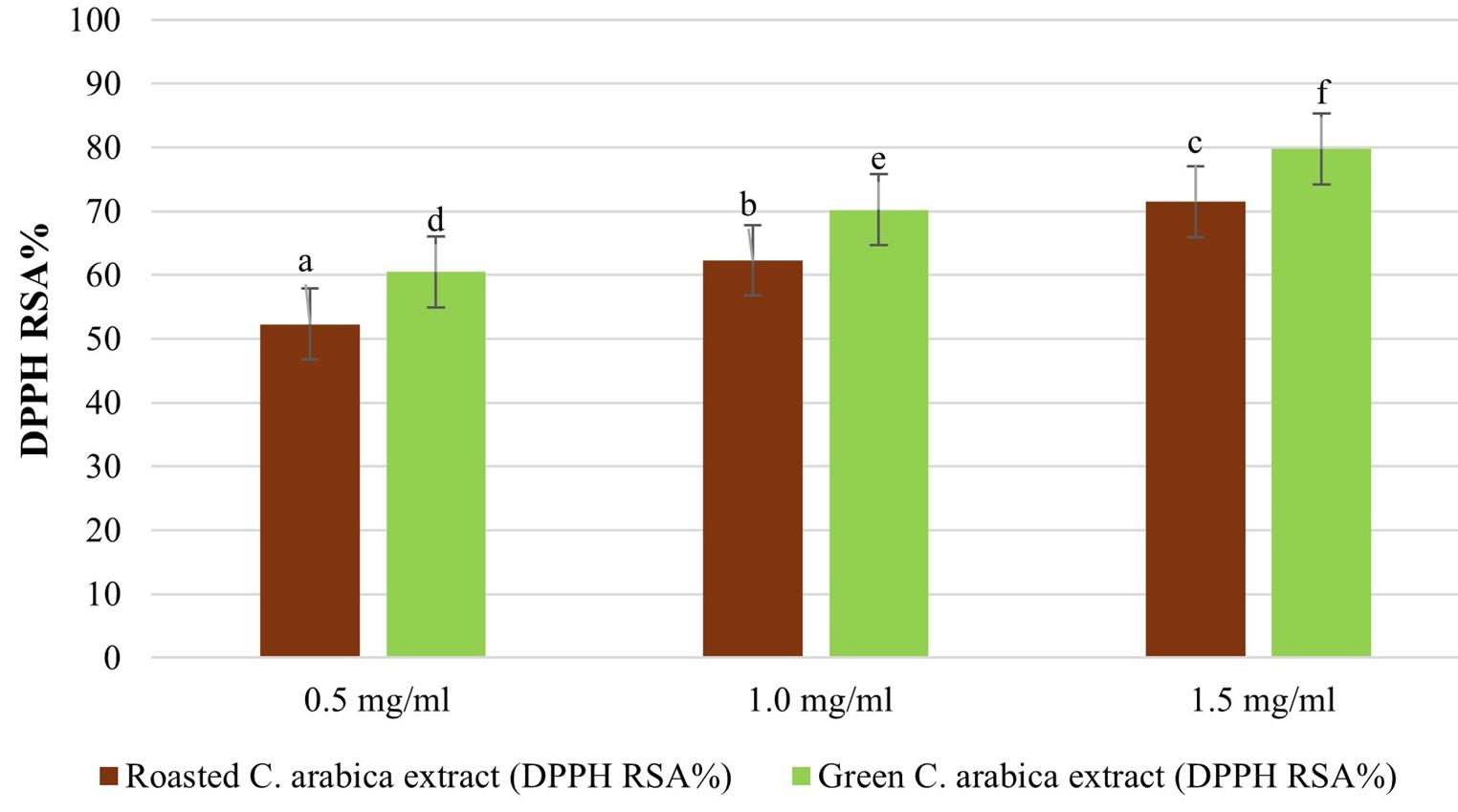
Effect of Coffee arabica-mediated CuNPs on mortality of mosquito larvae
Table 2 provides data on the effects of green and roasted C. arabica on A. aegypti larvae after 24 h. It included information on mortality, concentrations in ppm, standard deviation, total and mean mortality, as well as LC50 and LC90 values. The LC50 and LC90 concentrations for green C. arabica were 124.5 ppm and 456.5 ppm, respectively, while for roasted C. arabica, they were 73.5 ppm and 371.5 ppm, respectively. In addition, CuNPs concentrations ranging from 2 to 100 ppm caused mortality rates of 20% to 96.3% in exposed larvae, with corresponding LC50 and LC90 concentrations of 5.796 ppm and 36.595 ppm after 24 h. The chi-square value (df - 3) for green, roasted C. arabica and CuNPs were 6.482, 4.610 and 1.573, respectively. The results demonstrate a correlation between mosquito mortality and LC50.
Table 2. Larvicide of green and roasted C. arabica and CuNPs.
Effect of Coffee arabica-mediated CuNPs on morphology of Aedes aegypti
The findings of morphological analysis revealed the presence of damaged areas in the mid-gut region, characterized by pronounced pigmentation and darkening (Figure 3). Furthermore, the analysis identifies ruptured cuticles and deformed bodies, with a noticeable reduction in body size, particularly in the head and siphon.
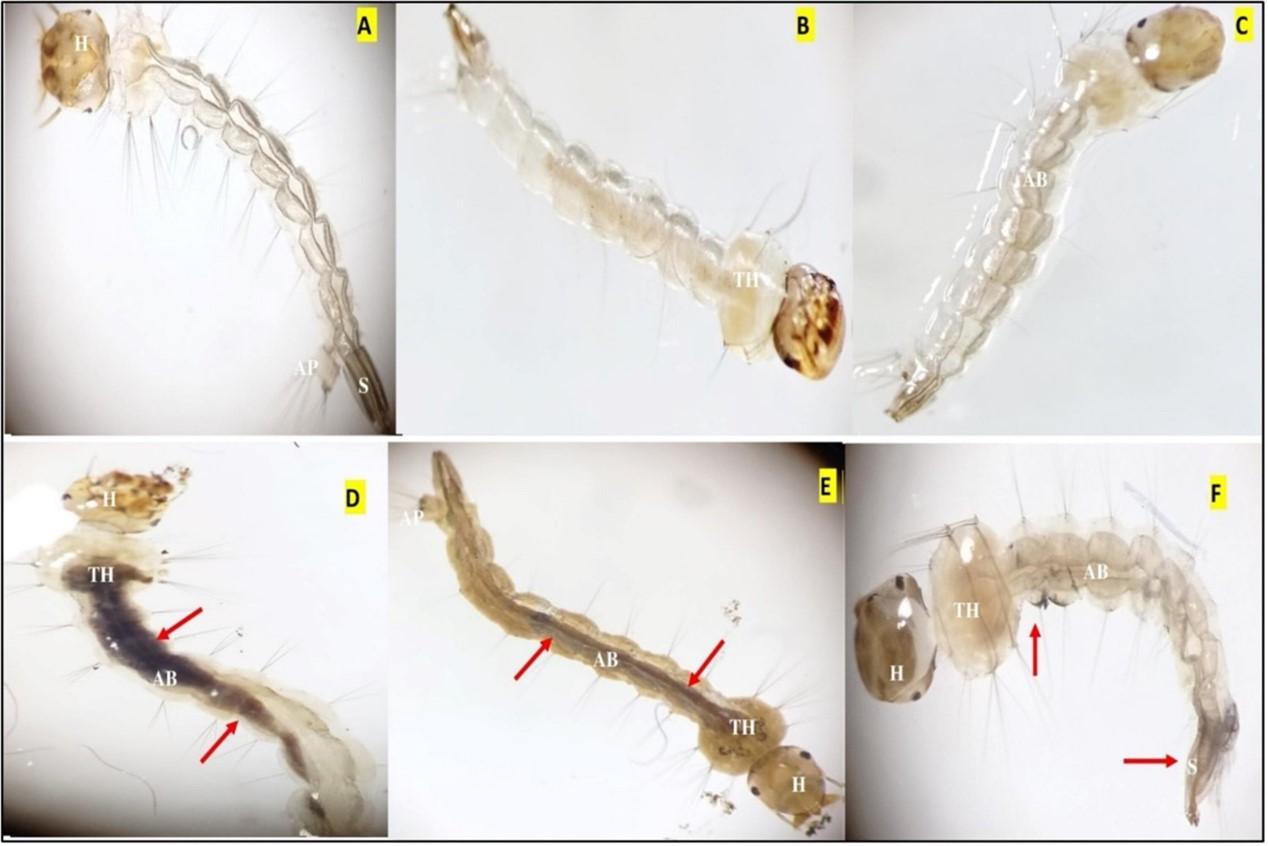
Effect of Coffee arabica-mediated CuNPs on histopathological alterations
In the current study, histological investigation was conducted as represented in Figure 4. The results revealed a significant level of tissue degradation in fourth instar Aedes aegypti larvae treated with CuNPs. Notably, the head region experienced complete tissue breakdown, while the stomach pouches in the larva's thorax region exhibited only minor changes. The cells in the treated larvae appeared similar to those in the control larvae, with intact nuclei, except for the presence of splits in the protective layer surrounding the gut and localized destruction of gut lining cells. The larva's digestive tract showed various indications of damage, including lesions. Additionally, the microvilli layer on the surface of mid-gut epithelial cells was noticeably thinner compared to the intact and tightly packed brush border observed in the gut epithelial cells of healthy larvae.
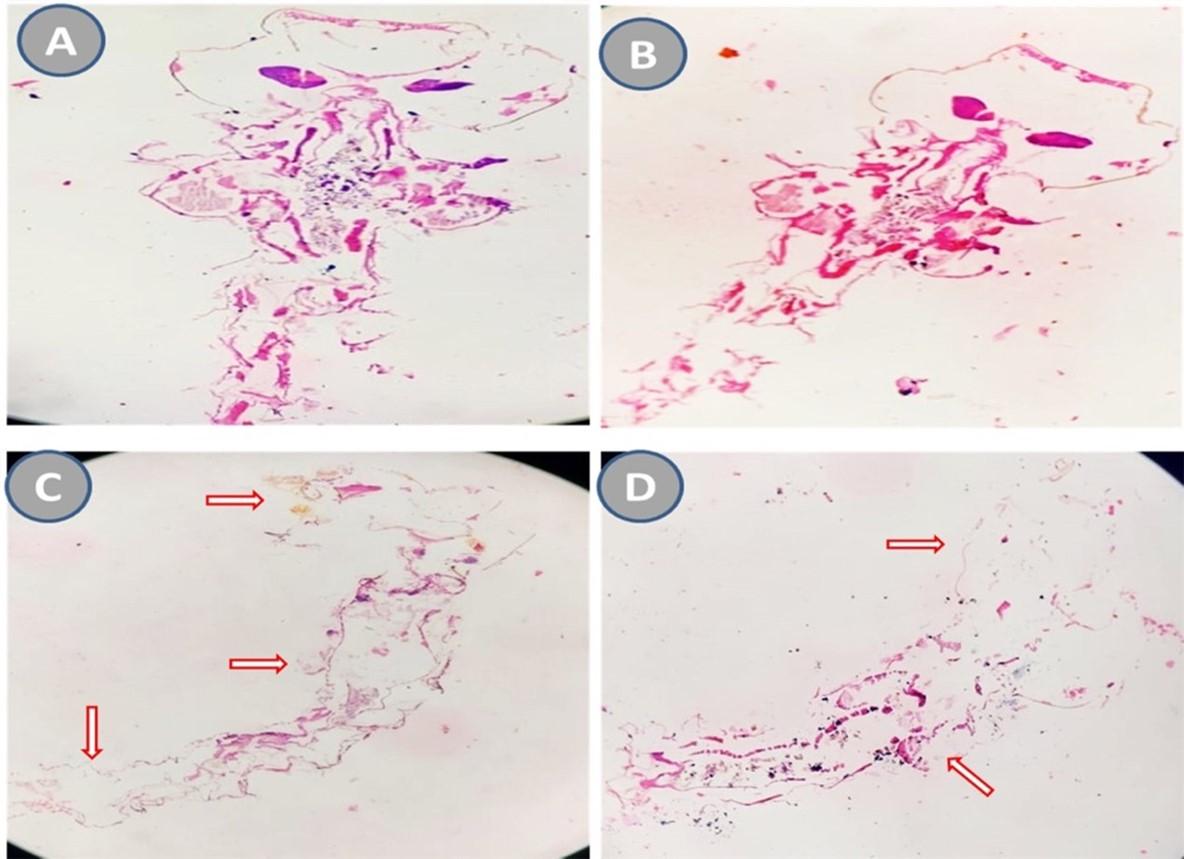
Effect of Coffee arabica-mediated CuNPs on ecotoxicological parameters
The study investigated the impact of varying concentrations of CuNPs on the survival of Artemia salina larvae. Results in Table 3 showed that higher concentrations of CuNPs led to increased larval mortality rates. The LC50 and LC90 values were determined to be 344.3 ppm and 1073.2 ppm, respectively. The findings suggest a significant relationship between CuNPs concentration and larval mortality.
Table 3. Toxicity study of CuNPs on Artemia salina.
DISCUSSION
CuNPs are formed through a synthesis process. Copper ions from the copper sulfate pentahydrate solution react with reducing agents in the Coffee arabica extract. The reduction process converts the ions into metallic copper atoms, which form the core of the nanoparticles. The core is surrounded by a shell composed of organic molecules from the extract, which stabilize the nanoparticles and control their size and dispersibility. The core-shell structure provides stability and unique properties to the CuNPs [28].
The anti-inflammatory, antioxidant, and potential health benefits of coffee are attributed to its components. Tannins, phenols, and flavonoids act as antioxidants, while caffeine promotes wakefulness. Saponins and terpenoids have anti-parasitic properties. With its abundant phytochemical content, coffee is a widely consumed beverage that may offer health advantages. This study revealed the presence of certain phytochemicals in Coffee arabica extract, consistent with findings from other research [29, 30].
Green coffee exhibits higher levels of total phenolic content and total flavonoid capacity compared to roasted coffee, which can be attributed to the breakdown of phenolic components during the roasting process. Both green and roasted coffee contain TPC and TFC. Although the concentrations may be slightly higher in green coffee, a statistically significant difference was observed (p < 0.000). However, it is worth noting that many individuals prefer the robust flavor profile of roasted coffee. Several researchers have conducted similar studies on TPC and TFC in green and roasted Arabica coffee, and their findings correspond relatively closely with our own [31, 32]. According to Sualeh et al. (2020), the average chlorogenic acid content was statistically significant and the caffeine content was not significant [33].
Antioxidants are important for protecting cells from damage caused by free radicals, which are associated with various chronic diseases. Therefore, the antioxidant properties of roasted and green Coffee arabica extracts may contribute to the potential health benefits attributed to coffee consumption. Similar to the findings of this investigation, a study by Jung et al. found that the DPPH radical scavenging activity decreased with increasing roasting time [32]. Additionally, Cho et al. have found that light roasting increases the DPPH radical scavenging activity of coffee extracts. On the other hand, as roasting temperatures increased, the DPPH radical scavenging activity reduced [34]. Consistent with Herawati et al., once the first crack is completed, the DPPH IC50 of coffee brewed from Robusta coffee beans increases as the roasting temperature rises [35]. Additionally, coffee has antioxidant properties in addition to its well-known toxicological effects, which makes it a good natural dietary supplement for beehives to increase their resistance to pesticides and other environmental stresses [36].
The present study observed that roasted coffee exhibited greater efficacy in terms of larvicidal effects when compared to green coffee. This finding aligns with previous research conducted in the field [37-39]. Thanasoponkul et al. (2023) investigated the impact of using 50 g/L of used coffee grounds and reported complete mortality of larvae within a span of 48 h. Similarly, Sharawi (2023) determined an effective concentration of Coffee arabica extract for this purpose. Furthermore, Tangtrakulwanich et al. (2022) conducted a study that demonstrated the inhibitory effects of lightly roasted used coffee grounds on larval survival. Mortality in mosquito larvae is attributed to alkaloid compounds, flavonoids, and tannins that hinder the insect's nervous system. The bitterness of coffee grounds can also cause irritation and disrupt digestion, leading to larval demise. Caffeine, found in coffee and other beverages, has been extensively studied for its toxicological effects on various organisms [39].
CuNPs have been found to have a more significant impact on mosquito larvae compared to coffee extract alone. To the best of our knowledge no available data on the larvicidal activity of CuNPs synthesized from Coffee arabica plants. Rajagopal et al. (2021b) demonstrated the larvicidal effects of CuNPs derived from Wrightia tinctoria extract on Aedes aegypti larvae, with an LC50 value of 0.0321 mg/ml. Similar outcomes were observed with Tridax procumbens, Artocarpus heterophyllus, and Metarhizium robertsii leaf extracts [40, 41, 42]. CuO/SiO2 nanoparticles synthesized from gum arabic extract showed larvicidal activity with an LC50 value of 4.17 mg/l. Cu/Ni and Cu/Co nanoparticles derived from palmyra palm fruit extract exhibited LC50 values of 18.50 and 16.076 ppm against Culex quinquefasciatus larvae, respectively [43-46]. CuNPs synthesized from Lantana camara leaf extracts were effective against Anopheles multicolor larvae, with an LC50 value of 12.6 ppm [47]. Aegle marmelos leaf extract-derived CuNPs demonstrated increased larvicidal effects against Anopheles stephensi, Aedes aegypti, and Culex quinquefasciatus, with LC50 values of 508.31, 538.25, and 582.73 ppm, respectively [48]. CuNPs derived from Achillea fragrantissima and Nigella sativa extracts exhibited larvicidal effects against Culex pipiens mosquitoes, with an LC50 value of 0.98 ppm [49]. The accumulation of CuNPs in the alimentary canal and their toxic effects on mosquito larvae contribute to their impact. Morphological changes, such as body shrinkage, darkening, pigmentation, and neck prolongation, have been observed in treated larvae as shown in Figure 3. Similar damage and abnormalities have been reported in other studies [50-52].
Corresponding to histopathology, the findings match the observations of other researchers who have suggested the impact of nanoparticles on mosquito tissue. According to Sandhu et al. [27, 53], exposing fourth instar larvae of Aedes aegypti to a ZnS-based hybrid nano emulsion resulted in the breakdown of various tissues and organs located in different regions. For instance, Mahmoud et al. [54] investigated the larvicidal potential of Piper nigrum extracts and observed significant histopathological alterations. The larva exhibited elongated cells with large vacuoles in the lining of the digestive tract. The protective layer surrounding the gut was torn, and the supporting tissue layer for the epithelial cells became detached. The surface projections of the epithelial cells appeared disorganized, and damage was observed in the muscular layer of the abdomen. Similarly, Raguvaran et al. [55] described the detrimental effects of silver nanoparticles on brush border cells and epithelial cells compared to untreated control larvae. The interaction between CuNPs and protein molecules in cells can hinder physiological enzymatic reactions in organisms, potentially leading to toxic reactions in insects.
In relation to ecotoxicological research, Vivekanandhan et al. (2021) observed decreased toxicity of CuNPs towards Artemia salina, with LC50 and LC90 values ranging from 293.9 to 980.2 μg/mL [42]. Another study by Kumaresn et al. (2015) found that environmentally feasible levels of CuO NP suspensions were not acutely toxic to Artemia salina larvae, but prolonged exposure resulted in significant toxicity [56]. The aggregation of CuO NPs into larger particles in seawater reduced their toxicity, and the effects varied with nanoparticle concentration. Cimen et al. (2020) found that the accumulation and elimination rates of copper and copper oxide nanoparticles in Artemia salina varied depending on the concentration and application time, with higher concentrations leading to increased mortality rates [57]. Khan et al. (2020) evaluated the toxicity of silver nanoparticles synthesized from Pinus wallichiana stem extract on Artemia salina. They observed mortality rates of 53%, 36%, and 30% at concentrations of 1000, 100, and 10 μg/mL, respectively, and a LD50 value of 717.8 for silver nanoparticles (AgNPs) on brine shrimp [58].
Kamaraj et al. (2023) found that the utilization of Cassia auriculata (CA) extract for synthesizing AgNPs resulted in low toxicity towards Artemia nauplii, with a mortality rate of 28.14% [59]. Pecoraro et al. (2021) investigated the toxicity of different metal nanoparticles (AuNPs, AgNPs, TiO2 NPs) on Artemia salina, focusing on their effects on cyst hatching and larval mortality. They observed that TiO2 NPs were non-toxic to both larvae and cysts, while AuNPs had toxic effects on hatching but did not impact larval development. AgNPs caused larval mortality and inhibited cyst hatching [60]. Ragavendran et al. (2023) conducted a recent study on the synthesis of zinc oxide nanoparticles (ZnO NPs) using Ficus racemosa leaf extract. The study found that these ZnO NPs exhibited low toxicity towards Artemia salina, suggesting their potential as environmentally friendly and versatile products [61]. Overall, these studies provide valuable insights into the safety and potential benefits of nanoparticles synthesized using plant extracts, paving the way for further exploration and utilization of this method in nanotechnology and related fields.
CONCLUSION
This study explored the larvicidal activity of Coffee arabica-synthesized CuNPs against Aedes aegypti mosquito larvae. The CuNPs were synthesized using Coffee arabica extract and characterized using advanced techniques. The extract contained bioactive compounds with antioxidant properties. Green coffee had a higher total phenolic content and total flavonoid capacity compared to roasted coffee. Green coffee extract displayed stronger antioxidant activity. CuNPs showed concentration-dependent mortality, with lower LC50 values compared to coffee extracts alone. Histological analysis revealed tissue degradation in treated larvae, particularly in the head region. CuNPs also had a detrimental effect on Artemia salina larvae, with higher concentrations resulting in increased mortality. The study suggests that Coffee arabica-synthesized CuNPs have strong larvicidal activity against Aedes aegypti mosquitoes, presenting potential for eco-friendly mosquito control strategies.
ACKNOWLEDGEMENT
The authors express their gratitude to the Hasanuddin University Mathematics and Natural Science Faculty (FMIPA) for their support in conducting this study, and also extend special thanks to the Medicine Science Faculty at Taiz University, Yemen, for providing laboratory facilities.
AUTHOR CONTRIBUTIONS
YA, AA, IW, PT, and HK collaborated as a team to execute the research plan, conduct practical research, collect data, and prepare the manuscript. The finalized manuscript has been reviewed and approved by each author.
CONFLICTS OF INTEREST
There is no conflict of interest among the authors.
References
- [1]Zeghoud S, Hemmami H et al. A review on biogenic green synthesis of ZnO nanoparticles by plant biomass and their applications. Materials Today Communications 2022; 33: 104747
- [2]Huq MA, Ashrafudoulla M et al. Green synthesis and potential antibacterial applications of bioactive silver nanoparticles: A review. Polymers 2022; 14: 742
- [3]Urnukhsaikhan E, Bold B-E et al. Antibacterial activity and characteristics of silver nanoparticles biosynthesized from Carduus crispus. Sci Rep 2021; 11: 21047
- [4]Malik S, Muhammad K et al. Nanotechnology: A revolution in modern industry. Molecules 2023; 28: 661
- [5]Mehtab A, Ahmed J et al. Rare earth doped metal oxide nanoparticles for photocatalysis: a perspective. Nanotechnology 2021; 33
- [6]Aslam M, Abdullah AZ et al. Recent development in the green synthesis of titanium dioxide nanoparticles using plant-based biomolecules for environmental and antimicrobial applications. Journal of Industrial and Engineering Chemistry 2021; 98: 1–16
- [7]Mazraedoost S, Masoumzade R et al. The role of nanoparticles for reactive oxygen species (ROS) in biomedical engineering. Advances in Applied NanoBio-Technologies 2021; 2: 24–36
- [8]Priya SS, Vasantha-Srinivasan P et al. Bioactive Molecules Derived from Plants in Managing Dengue Vector Aedes aegypti (Linn.). Molecules 2023; 28: 2386
- [9]Biswas P, Polash SA et al. Advanced implications of nanotechnology in disease control and environmental perspectives. Biomedicine & Pharmacotherapy 2023; 158: 114172
- [10]Patil S, Chandrasekaran R. Biogenic nanoparticles: a comprehensive perspective in synthesis, characterization, application and its challenges. J Genet Eng Biotechnol 2020; 18: 67
- [11]Chen L, Min J et al. Copper homeostasis and cuproptosis in health and disease. Signal transduction and targeted therapy 2022; 7: 378
- [12]Hasanin M, Al Abboud MA et al. Ecofriendly synthesis of biosynthesized copper nanoparticles with starch-based nanocomposite: antimicrobial, antioxidant, and anticancer activities. Biological Trace Element Research 2021; 1–14
- [13]Lange A, Grzenia A et al. Silver and copper nanoparticles inhibit biofilm formation by mastitis pathogens. Animals 2021; 11: 1884
- [14]Mani M, Sundararaj AS et al. Rapid synthesis of copper nanoparticles using Nepeta cataria leaves: An eco-friendly management of disease-causing vectors and bacterial pathogens. Green Processing and Synthesis 2023; 12: 20230022
- [15]Alahdal FA, Qashqoosh MT et al. Green synthesis and characterization of copper nanoparticles using Phragmanthera austroarabica extract and their biological/environmental applications. Sustainable Materials and Technologies 2023; 35: e00540
- [16]Al-Jubouri AK, Al-Saadi NH et al. Green synthesis of copper nanoparticles from myrtus communis leaves extract: Characterizattion, antioxidant and catalytic activity. Iraqi journal of agricultural sciences 2022; 53: 471–486
- [17]Al-Khafaji MAA, Al-Refai’a RA et al. Green synthesis of copper nanoparticles using Artemisia plant extract. Materials Today: Proceedings 2022; 49: 2831–2835
- [18]Nieto-Maldonado A, Bustos-Guadarrama S et al. Green synthesis of copper nanoparticles using different plant extracts and their antibacterial activity. Journal of Environmental Chemical Engineering 2022; 10: 107130
- [19]Wu C-S, Chiang H-M et al. Prospects of Coffee Leaf against SARS-CoV-2 Infection. Int J Biol Sci 2022; 18: 4677–4689
- [20]Wu PY, Huang CC et al. Alleviation of ultraviolet B-induced photodamage by Coffea arabica extract in human skin fibroblasts and hairless mouse skin. International Journal of Molecular Sciences 2017; 18
- [21]Drago A, Vettore S et al. Efficacy of used coffee grounds as larvicide against Aedes albopictus (Skuse, 1894) and Ae. aegypti Linné, 1762 (Diptera: Culicidae). Journal of the European Mosquito Control Association 2021; 39: 27–30
- [22]Kaitana Y, Kurnia N et al. The potentials of Pangi leaf extract for Aedes spp. mosquito control. J Adv Biotechnol Exp Ther 2023; 6: 133
- [23]Lestari W, Hasballah K et al. Antioxidant and phytometabolite profiles of ethanolic extract from the cascara pulp of Coffea arabica collected from Gayo Highland: A study for potential photoaging agent. F1000Res 2023; 12: 12
- [24]Nurcholis W, Aisyah SI et al. The Analysis of Morphological Diversity and Polyphenols Content of Celosia cristata in M2 Population Induced by Ethyl Methane Sulphonate: Genetic diversity of mutated C. cristata. Journal of Tropical Life Science 2023; 13: 115–122
- [25]Sharma KR, Khanal N. Comparative investigation on antihyperglycemic and antioxidant activity of Zingiber officinale growing in different regions of Nepal. Sci World 2022; 15: 33–43
- [26]WHO. WHO (2005) Guidelines for laboratory and field testing of mosquito larvicides world health organization communicable disease control, prevention and eradication who pesticide. Geneva 1: 25 WHO. CDS/WHOPES/GCDPP/2005.13. https://apps. who. int/iris/handle/10665/69101 …, 2019
- [27]Sandhu KK, Vashishat N et al. Formulation of eucalyptus oil-zinc sulphide hybrid nanoemulsion and evaluation of its larvicidal potential against Aedes aegypti. African Entomology 2023; 31: 1–8
- [28]Abdulwahab Y, Ahmad A et al. Green synthesis of copper nanoparticles mediated from coffee arabica seeds extract. Rasayan Journal of Chemistry 2023; 16
- [29]Alemu MA, Birhanu Wubneh Z et al. Antidiarrheal Effect of 80% Methanol Extract and Fractions of the Roasted Seed of Coffea arabica Linn (Rubiaceae) in Swiss Albino Mice. Evidence-Based Complementary and Alternative Medicine 2022; 2022: e9914936
- [30]Fatmawati S, Sjahid LR et al. Total Phenolic, Total Flavonoid Content and in vitro Sun Protection Factor test of Arabica Coffee Leaves Extract (Coffea arabica L). Journal of Science and Technology Research for Pharmacy 2021; 1: 57–66
- [31]Alnsour L, Issa R et al. Quantification of total phenols and antioxidants in coffee samples of different origins and evaluation of the effect of degree of roasting on their levels. Molecules 2022; 27: 1591
- [32]Jung S, Gu S et al. Effect of roasting degree on the antioxidant properties of espresso and drip coffee extracted from Coffea arabica cv. Java. Applied Sciences 2021; 11: 7025
- [33]Sualeh A, Tolessa K et al. Biochemical composition of green and roasted coffee beans and their association with coffee quality from different districts of southwest Ethiopia. Heliyon 2020; 6
- [34]Cho AR, Park KW et al. Influence of Roasting Conditions on the Antioxidant Characteristics of Colombian Coffee (Coffea arabica L.) Beans. Journal of Food Biochemistry 2014; 38: 271–280
- [35]Herawati D, Giriwono PE et al. Critical roasting level determines bioactive content and antioxidant activity of Robusta coffee beans. Food Sci Biotechnol 2019; 28: 7–14
- [36]Balieira KVB, Mazzo M et al. Imidacloprid-induced oxidative stress in honey bees and the antioxidant action of caffeine. Apidologie 2018; 49: 562–572
- [37]Sharawi S. The toxic effect of some traditional herbs in the Kingdom of Saudi Arabia against Aedes aegypti larvae as a safety method for control. International Journal of Mosquito Research 2023; 10: 15–20
- [38]Thanasoponkul W, Changbunjong T et al. Spent Coffee Grounds and Novaluron Are Toxic to Aedes aegypti (Diptera: Culicidae) Larvae. Insects 2023; 14: 564
- [39]Tangtrakulwanich K, Suwannawong B et al. The Comparative Study of Arabica Used Coffee Grounds and Temephos in Controlling the Aedes aegypti Larvae. Journal of Food Science and Agricultural Technology (JFAT) 2022; 6: 1–5
- [40]Muthamil Selvan S, Vijai Anand K et al. Green synthesis of copper oxide nanoparticles and mosquito larvicidal activity against dengue, zika and chikungunya causing vector Aedes aegypti. IET Nanobiotechnology 2018; 12: 1042–1046
- [41]Sharon EA, Velayutham K et al. Biosynthesis of Copper Nanoparticles using Artocarpus heterophyllus against Dengue Vector Aedes aegypti. IJLSSR 2018; 4: 1872–1879
- [42]Vivekanandhan P, Swathy K et al. Insecticidal efficacy of microbial-mediated synthesized copper nano-pesticide against insect pests and non-target organisms. International Journal of Environmental Research and Public Health 2021; 18
- [43]Abba E, Shehu Z et al. Synthesis, characterization and application of cuo@ sio2 nanocomposite using gum arabic: a bio-friendly control measure against lymphatic filariasis vector. Plant cell biotechnology and molecular biology 2021; 99–108
- [44]Danbature WL, Shehu Z et al. Green synthesis, characterization and larvicidal activity of Cu/Ni bimetallic nanoparticles using fruit extract of Palmyra palm. International Journal of Chemistry and Materials Research 2020; 8: 20–25
- [45]Shehu Z, Danbature W et al. Synthesis, characterization and application of cuo@sio 2 nanocomposite using gum arabic: a bio-friendly control measure against lymphatic filariasis vector. Plant Cell Biotechnology and Molecular Biology 2021; 22: 99–108
- [46]Shehu Z, Danbature WL et al. Green synthesis and nanotoxicity assay of Copper-Cobalt bimetallic nanoparticles as a novel nanolarvicide for mosquito larvae management. The International Journal of Biotechnology 2020; 9: 99–104
- [47]Abd El Hafiz Hassanain N, Shaapan M et al. Comparison Between Insecticidal Activity of Lantana camara Extract and its Synthesized Nanoparticles Against Anopheline mosquitoes. Pakistan Journal of Biological Sciences: PJBS 2019; 22: 327–334
- [48]Hassan SE-D, Fouda A et al. Endophytic actinomycetes Streptomyces spp mediated biosynthesis of copper oxide nanoparticles as a promising tool for biotechnological applications. J Biol Inorg Chem 2019; 24: 377–393
- [49]Al-Ghabban A, Eldiasty J. Green Synthesis of Copper Oxide Nanoparticle by Using Achillea fragrantissima and Nigella sativa Extracts and Their Effects as Larvicidal, Molluscicidal and Antimicrobial Agents. Egyptian Academic Journal of Biological Sciences, E Medical Entomology & Parasitology 2022; 14: 127–148
- [50]Dhavan PP, Jadhav BL. Eco-friendly approach to control dengue vector Aedes aegypti larvae with their enzyme modulation by Lumnitzera racemosa fabricated zinc oxide nanorods. SN Appl Sci 2020; 2: 843
- [51]Mahyoub JA. Biological effects of synthesized silver nanoparticles using Dodonaea viscosa leaf extract against Aedes aegypti (Diptera: Culicidae). J Entomol Zool Stud 2019; 7: 827–832
- [52]Sultana N, K. Raul P et al. Bio-nanoparticle assembly: a potent on-site biolarvicidal agent against mosquito vectors. RSC Advances 2020; 10: 9356–9368
- [53]Sandhu KK, Vashishat N et al. Larvicidal potential of copper sulphide nano aqua dispersions against Aedes aegypti (Linnaeus). African Entomology 2022; 30: 1–5
- [54]Mahmoud DM, Abd El-Bar MM et al. Larvicidal potential and ultra-structural changes induced after treatment of Culex pipiens L.(Diptera: Culicidae) larvae with some botanical extracted oils. synthesis 2019; 12: 15–18
- [55]Raguvaran K, Kalpana M et al. Larvicidal, antioxidant and biotoxicity assessment of (2-(((2-ethyl-2 methylhexyl) oxy) carbonyl) benzoic acid isolated from Bacillus pumilus against Aedes aegypti, Anopheles stephensi and Culex quinquefasciatus. Archives of Microbiology 2022; 204: 650
- [56]Kumaresn K, Parthiban D et al. Toxicity Effect of Copper oxide Nanoparticles on Artemia salina. Research Journal of Pharmacology and Pharmacodynamics 2015; 7: 53–60
- [57]Cimen ICC, Danabas D et al. Comparative effects of Cu (60–80 nm) and CuO (40 nm) nanoparticles in Artemia salina: Accumulation, elimination and oxidative stress. Science of The Total Environment 2020; 717: 137230
- [58]Khan N, Khan I et al. Pinus wallichiana-synthesized silver nanoparticles as biomedical agents: in-vitro and in-vivo approach. Green Chemistry Letters and Reviews 2020; 13: 69–82
- [59]Kamaraj C, Ragavendran C et al. Green synthesis of silver nanoparticles from Cassia Auriculata: Targeting antibacterial, antioxidant activity, and evaluation of their possible effects on saltwater microcrustacean, Artemia Nauplii (non-target organism). Science of The Total Environment 2023; 861: 160575
- [60]Pecoraro R, Scalisi EM et al. Artemia salina: A microcrustacean to assess engineered nanoparticles toxicity. Microscopy Research and Technique 2021; 84: 531–536
- [61]Ragavendran C, Kamaraj C et al. Eco-friendly approach for ZnO nanoparticles synthesis and evaluation of its possible antimicrobial, larvicidal and photocatalytic applications. Sustainable Materials and Technologies 2023; 36: e00597